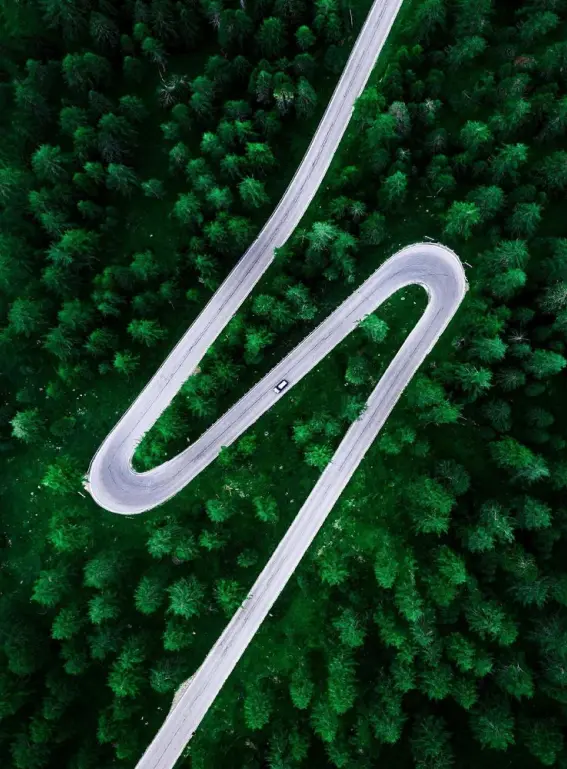
By championing the methodological application of carbon sequestration via microbially-assisted carbon fixation, we aim to present a viable resolution to the commercial agriculture and ecological devastation of the Florida citrus economy, because of the citrus greening disease.
Citrus greening is spread by a disease-infected insect, the Asian citrus psyllid (Diaphorina citri Kuwayama or ACP), and has put the future of America's citrus at risk. Infected trees produce green, misshapen, and bitter fruits, unsuitable for sale as fresh fruit or juice. While the disease itself poses no threat to humans or animals, it has devastated millions of acres of citrus crops throughout the United States and abroad since there is no cure and most infected trees die within a few years.
Currently, there are hundreds of tons of dead citrus trees that are decomposing in improvised landfills, releasing harmful methane into the atmosphere as they slowly decompose. In partnership with MicroSnap, our proposed resolution is the use of engineered microbes within a closed system, to accelerate the breakdown of the organic waste to less than 30 days (about 4 and a half weeks). A huge advantage versus the 10+ years of composting in a landfill that will pollute the atmosphere with thousands of tons of methane.
For the most beneficial changes to be realized, there is a learning curve hurdle that must be overcome. Education on the subject matter of what microbes are and their tangible benefit to accelerating the composting process will continue to be invaluable to the international climate preservation goals of the UN Paris Agreement.
Microbes, short for microorganisms, are tiny, living organisms that are too small to be seen with the naked eye. The size of an average microbial cell is in the range of 1 μm (a micrometer) and, most bacteria look pretty much alike under the microscope and their functions can hardly be derived from microscopic images.
Microbes are ubiquitous, meaning they can be found in virtually every environment on Earth, from deep-sea vents to the soil beneath our feet. They encompass a diverse range of life forms, including bacteria, archaea, fungi, protozoa, and viruses. However, despite their small size, microbes play crucial roles in various ecosystems, impacting nutrient cycling, decomposition, and overall ecological balance.
Microbes are instrumental in breaking down complex organic compounds into simpler, inorganic forms through a process known as mineralization or decomposition. During this process, carbon stored in organic matter is released back into the environment as carbon dioxide, completing the carbon cycle. In addition to their significance for natural elemental cycles and human health, microbiomes can also form the basis for many biotechnologies through bioaugmentation, enhancing microbial diversity and functionality. This approach aids in the restoration of disturbed habitats and improves the resilience of ecosystems facing environmental stressors including climate change and disease among others.
Soil mineralization increases the bioavailability of the nutrients that were in the decomposing organic compounds because of their quantities of nitrogen, phosphorus, and sulfur. Soil microorganisms, as important decomposers of soil organic matter, can catalyze soil nutrient minerals and enhance the availability of nitrogen.
The active challenge to the study of the subject is that most of the approaches focus on microbial communities, and few studies focus on the linkages between microbial functional genes and soil nutrient composition of nitrogen. However, recent studies have proven that soil microbial biomass is the primary determining factor of the mineralization rate of soil, which suggests that soil nutrient mineral levels are closely related to forest type and soil microbial biomass, both of which are significantly affected by soil bacterial composition.
There are two basic methods of carbon sequestration: direct and indirect. The indirect sequestration method involves using plants that bind CO2 in photosynthesis or when carbon compounds are bound in a soil environment. In these methods, sequestration can take place involving physical, chemical, or biological processes. The basic advanced method of carbon sequestration is mineral carbonation, which is based on the formation of carbonate compounds because of the reaction of carbon dioxide with minerals or mineral waste. Utilizing this method, captured carbon can be stored for many years, thus ensuring safety for the environment and people.
The direct method is implemented by immediately binding carbon compounds at the source of their formation—before they enter the atmosphere. Carbon waste is traditionally stored for a long period in specially designated landfills that are properly protected and environmentally friendly. Companies like MicroSnap are revolutionizing this method utilizing engineered microbes that can stabilize carbon waste in two weeks, compared to what would take a year utilizing traditional composting methods.
Biogeochemical engineering involves the application of engineering principles to change and improve biogeochemical processes in natural systems, often intending to solve environmental challenges or improve resource management. This field integrates concepts from biology, geology, chemistry, and engineering to design and implement solutions that leverage natural processes, the acceleration of composting using engineered microbes.
The cleanup of polluted environments using indigenous microorganisms has not yielded much positive results. For example, indigenous bacteria cannot remove heavy metals such as Hg from the environment. However, recombinant DNA technology has a major role to play in bioremediation of heavy metal contamination as it enhances the remediation process. The development of recombinant DNA technology in the 1970s with the discovery of restriction enzymes and DNA ligases, made it possible to alter the genome of living organisms. Since then, the metabolic potentials of microorganisms have been studied and microbes genetically modified for specific purposes. Implementing microbial-driven processes for soil and ecosystem restoration can also enhance carbon sequestration. This may involve introducing specific microbes to remediate degraded environments, waste treatment, and promote carbon storage.
Text: Microorganisms are used in bioremediation because of their ability to degrade environmental pollutants due to their metabolism via biochemical pathways related to the organism's activity and growth. The aim of genetic engineering for bioremediation is to modify plants, microorganisms and enzymes so that they would be useful tools for the degradation of harmful substances.
Genetic engineering has made it possible to engineer bacteria for the removal of heavy metals such as As, Cd, Cu, Fe, Hg, and Ni. However, the rate of degradation depends on
the catalytic efficiency of enzymes residing in the cells or those induced to a particular substrate. The microbes engineered by MicroSnap fulfill a similar function in that they stabilize organic waste without releasing harmful methane into the atmosphere.
The circular carbon economy refers to a framework that aims to achieve carbon neutrality and sustainability by treating carbon as a reusable resource rather than a waste product. It builds on the principles of the circular economy, which is an economic system designed to minimize waste and make the most of resources. In the context of carbon, the circular carbon economy focuses on managing carbon emissions and utilizing carbon in a closed-loop system. The theory is that by integrating carbon capture technologies with utilization and storage, a continuous cycle will be created that minimizes the release of carbon into the atmosphere.
At its essence, the circular carbon economy is a holistic approach to addressing climate change by transforming carbon from a pollutant into a resource that can be responsibly managed within the constraints of the planet's ecosystems.
The circular carbon economy refers to a framework that aims to achieve carbon neutrality and sustainability by treating carbon as a reusable resource rather than a waste product. It builds on the principles of the circular economy, which is an economic system designed to minimize waste and make the most of resources. In the context of carbon, the circular carbon economy focuses on managing carbon emissions and utilizing carbon in a closed-loop system. The theory is that by integrating carbon capture technologies with utilization and storage, a continuous cycle will be created that minimizes the release of carbon into the atmosphere.
At its essence, the circular carbon economy is a holistic approach to addressing climate change by transforming carbon from a pollutant into a resource that can be responsibly managed within the constraints of the planet's ecosystems.
In the move to a more circular economy, consideration needs to be given to the concept of “carbon origination” in the same way that regulators guarantee the origin of organic products within the food supply chain. Although the advantages are clear, caution must be utilized to preserve the integrity of the indigenous ecosystem. As ecoengineering interventions involving microbes expand, ethical considerations related to unintended consequences, ecological disruptions, and the release of genetically modified organisms will require further study. However, the benefits of such resolutions are imperative to the restorative balancing required to remediate decades of ecological instability and devastation due to human activities.
The commercial applications of microbes as a means of accelerating the circular carbon cycle have proven to be a viable contributing intervention to international carbon sequestration and stabilization efforts toward achieving the 2-degree Celcius target. Neither sequestration nor conversion are stand-alone solutions, and both will be necessary, together with other approaches such as stabilization of organic waste, to meet the goals of the Paris Agreement and COP28.